Flap (aircraft)
Flaps are normally hinged surfaces mounted on the trailing edges of the wings of a fixed-wing aircraft to reduce the speed an aircraft can be safely flown at and to increase the angle of descent for landing without increasing air speed. They shorten takeoff and landing distances as well as improving climb rate. It does this by increasing the amount of lift generated, by lowering the stall speed and increasing the drag.
Extending flaps primarily increases the camber or curvature of the wing airfoil raising the maximum lift coefficient - or the lift a specific wing section may generate. This allows the aircraft to generate as much lift but at a lower speed, reducing the stalling speed of the aircraft, or the minimum speed at which the aircraft will maintain flight. Extending flaps increases drag which can be beneficial during approach and landing because it slows the aircraft. On some aircraft a useful side effect of flap deployment is a decrease in aircraft pitch angle which improves the pilot's view of the runway over the nose of the aircraft during landing, however the flaps may also cause pitchup, depending on the type of flap and the location of the wing.
There are many different types of flaps used, with the specific choice depending on the size, speed and complexity of the aircraft they are to be used on, as well as the era in which the aircraft was designed. Plain flaps, slotted flaps, and Fowler flaps are the most common. Flaps used on the leading edge of the wings of many jet airliners are called Krueger flaps. The Fowler, Fairey-Youngman and Gouge types of flap increase the planform area of the wing in addition to changing the camber. The larger lifting surface reduces wing loading and allows the aircraft to generate more lift at a given speed and reduces stalling speed but although the effect is similar to increasing the lift coefficient, raising the planform area of the wing does not necessarily change the lift coefficient which is dependent on the camber, not the chord.
Physics explanation
The general airplane lift equation demonstrates these relationships:
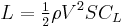
where:
- L is the amount of Lift produced,
is the air density,
- V is the indicated airspeed of the airplane or the Velocity of the airplane, relative to the air
- S is the planform area or Surface area of the wing and
is the lift coefficient which is determined by the camber of the airfoil used, the chord of the wing and the angle at which the wing meets the air (or angle of attack).
Here, it can be seen that increasing the area (S) and lift coefficient (
) allow a similar amount of lift to be generated at a lower airspeed (V).
Extending the flaps also increases the drag coefficient of the aircraft. Therefore, for any given weight and airspeed, flaps increase the drag force. Flaps increase the drag coefficient of an aircraft because of higher induced drag caused by the distorted spanwise lift distribution on the wing with flaps extended. Some flaps increase the planform area of the wing and, for any given speed, this also increases the parasitic drag component of total drag.
Flaps during takeoff
Depending on the aircraft type, flaps may be partially extended for takeoff. When used during takeoff, flaps trade runway distance for climb rate - using flaps reduces ground roll and the climb rate. The amount of flap used on takeoff is specific to each type of aircraft, and the manufacturer will suggest limits and may indicate the reduction in climb rate to be expected. The Cessna 172 Pilot Operating Handbook recommends not using any flaps on takeoff unless the ground is rough or soft.
Flaps during landing
Flaps may be fully extended for landing to give the aircraft a lower stalling speed so the approach to landing can be flown more slowly, which also allows the aircraft to land in a shorter distance. The higher lift and drag associated with fully extended flaps allows a steeper and slower approach to the landing site but imposes handling difficulties in aircraft with very low wing loading (the ratio between the wing area and the weight of the aircraft). Winds across the line of flight, known as crosswinds, cause the windward side of the aircraft to generate more lift and drag, causing the aircraft to roll, yaw and pitch off its intended flight path, and as a result many light aircraft have limits on how strong the crosswind can be while using flaps. Furthermore, once the aircraft is on the ground, the flaps may decrease the effectiveness of the brakes since the wing is still generating lift and preventing the entire weight of the aircraft from resting on the tires, thus increasing stopping distance, particularly in wet or icy conditions. Usually the pilot will raise the flaps as soon as possible to prevent this from occurring.
Maneuvering flaps
Some gliders not only use flaps when landing but also in flight to optimize the camber of the wing for the chosen speed. When thermalling, flaps may be partially extended to reduce the stalling speed so that the glider can be flown more slowly and thereby turn in a smaller circle to make best use of the core of the thermal. At higher speeds a negative flap setting is used to reduce the nose-down pitching moment. This reduces the balancing load required on the horizontal stabilizer, which in turn reduces the trim drag associated with keeping the glider in longitudinal trim. Negative flap may also be used during the initial stage of an aerotow launch and at the end of the landing run in order to maintain better control by the ailerons.
Like gliders, some fighters also use special flaps to improve maneuverability during air combat, allowing the fighter to bleed off speed quickly, allowing for much tighter turns. The flaps used for this must be designed specifically to handle the greater stresses as most flaps have a maximum speed at which they can be deployed.
Types of flaps
- Plain flap: the rear portion of airfoil rotates downwards on a simple hinge mounted at the front of the flap.[1] Used in this form as early as 1917 (during World War I) on the widely produced Breguet 14 and possibly earlier on experimental types.[2] Due to the greater efficiency of other flap types, the plain flap is normally only used where simplicity is required. A modern variation on the plain flap exploits the ability of composites to be designed to be rigid in one direction, while flexible in another. When such a material forms the skin of the wing, its camber can be altered by the geometry of the internal supporting structure, allowing such a surface to be used either as a flap or as an aileron. While most currently use a complex system of motors and actuators, the simplest such installation uses ribs that resemble bent carrots - when the bend is nearly horizontal, there is no deflection, but when the carrot is rotated so the bend is downward, the camber of the airfoil is changed in the same manner as on a plain flap.
- Split flap: the rear portion of the lower suface of the airfoil hinges downwards from the leading edge of the flap, while the upper surface stays immobile.[3] Like the plain flap, this can cause large changes in longitudinal trim, pitching the nose either down or up, and tends to produce more drag than lift. At full deflection, a split flaps acts much like a spoiler, producing lots of drag and little or no lift. It was invented by Orville Wright and James M. H. Jacobs in 1920 but only became common in the 1930s but was quickly superseded. The Douglas DC-3 & C-47 used a split flap.
- Slotted flap: a gap between the flap and the wing forces high pressure air from below the wing over the flap helping the airflow remain attached to the flap, increasing lift compared to a split flap.[4] Additionally, lift across the entire chord of the primary airfoil is greatly increased as the velocity of air leaving its trailing edge is raised, from the typical non-flap 80% of freestream, to that of the higher-speed, lower-pressure air flowing around the leading edge of the slotted flap.[5] Any flap that allows air to pass between the wing and the flap is considered a slotted flap. The slotted flap was a result of research at Handley-Page, a variant of the slot and dates from the 1920s but wasn't widely used until much later. Some flaps use multiple slots to further boost the effect.
- Fowler flap: split flap that slides backwards flat, before hinging downwards, thereby increasing first chord, then camber.[6] The flap may form part of the uppersurface of the wing, like a plain flap, or it may not, like a split flap but it must slide rearward before lowering. It may provide some slot effect but this is not a defining feature of the type.[7] Invented by Harlan D. Fowler in 1924, and tested by Fred Weick at NACA in 1932. They were first used on the Martin 146 prototype in 1935, and in production on the 1937 Lockheed Electra,[8] and is still in widespread use on modern aircraft, often with multiple slots. As mentioned under the plain flaps, variable geometry wings are making a comeback, and an F-111 was modified with such a system that acted as fowler flaps by NASA for trials on the AFTI/F-111 Mission Adaptive Wing.
- Junkers Flap: a slotted plain flap where the flap is fixed below the trailing edge of the wing, rotating about its forward edge.[9] When not in use it has more drag than other types but is more effective at creating additonal lift than a plain or split flap, while retaining their mechanical simplicity. Invented by O. Mader at Junkers in the late 1920s, it was widely used on the Junkers Ju 52, though it can be found on many modern ultralights.
- Gouge flap: a type of split flap that slides backwards along curved tracks that force the trailing edge downwards, increasing chord and camber without affecting trim or requiring any additional mechanisms.[10] It was invented by Arthur Gouge for Short Brothers in 1936 and used on the Short Empire and Short Sunderland flying boats which used the very thick Shorts A.D.5 airfoil. Short Brothers may have been the only company to use this type.
- Fairey-Youngman flap: drops down (becoming a Junkers Flap) before sliding aft and then rotating up or down. Fairey was one of the few exponents of this design, which was used on the Fairey Firefly and Fairey Barracuda. When in the extended position, it could be angled up (to a negative angle of incidence) so that the aircraft could be dived vertically without needing excessive trim changes.
- Zap Flap or commonly but incorrectly Zapp Flap: Invented by Edward F. Zaparka while he was with Berliner/Joyce and tested on a General Aircraft Corporation Aristocrat in 1932 and on other types periodically thereafter, but it saw little use on production aircraft other than on the Northrop P-61 Black Widow. The leading edge of the flap is mounted on a track, while a point at mid chord on the flap is connected via an arm to a pivot just above the track. When the flap's leading edge moves aft along the track, the triangle formed by the track, the shaft and the surface of the flap (fixed at the pivot) gets narrower and deeper, forcing the flap down.[11]
- Krueger flap: hinged flap which folds out from under the wing's leading edge while not forming a part of the leading edge of the wing when retracted. This increases the camber and thickness of the wing, which in turn increases lift and drag.[12][13] This is not the same as a leading edge droop flap, as that is formed from the entire leading edge.[14] Invented by Werner Krüger in 1943 and evaluated in Goettingen,[15] Krueger flaps are found on many modern swept wing airliners.
- Leading edge droop: entire leading edge of the wing rotating downwards,[16] effectively increasing camber but slightly reducing chord. Most commonly found on fighters with very thin wings unsuited to other leading edge high lift devices.
- Gurney flap: A small fixed perpendicular tab of between 1 and 2% of the wing chord, mounted on the high pressure side of the trailing edge of an airfoil. It was named for racing car driver Dan Gurney who rediscovered it in 1971, but has since used on some helicopters such as the Sikorsky S-76B to correct control problems without having to resort to a major redesign. It boosts the efficiency of even basic theoretical airfoils (made up of a triangle and a circle overlapped) to the equivalent of a conventional airfoil. The principle was discovered in the 1930s but was never proceeded with and was then forgotten.
- Blown flaps: also known as Boundary Layer Control Systems, are systems that blow engine air over the upper surface of any of the previously mentioned types of flap to improve lift characteristics. Two types exist - the original type blew air out of channels or holes in the surface of the flap, while newer systems simply blow engine exhaust over the top of the flap. These require ample reserves of power and are maintenance intensive thus limiting their use but they provide lots of lift at low airspeeds. Although invented by the British, the first production aircraft with blown flaps was the Lockheed F-104 Starfighter. The later type was trialled on the Boeing YC-14 in 1976.
- Controls that look like flaps but are not:
- Handley Page leading edge slats/slots may be confused for flaps but are mounted on the top of the wings' leading edge and while they may be either fixed or retractable, when deployed they provide a slot or gap under the slat to force air against the top of the wing which is absent on a Krueger flap. Other types of flaps may be equipped with one or more slots to increase their effectiveness, a typical setup on many modern airliners. These are known as slotted flaps as described above.
-
- Spoilers may also be confused for flaps but are intended solely to create drag and not lift. A spoiler is much larger than a Gurney flap, and can be retracted.
-
- Ailerons are similar to flaps (and work the same way) but are intended to provide lateral control, rather than to change the lifting characteristics of both wings together, and so operate differentially - when an aileron on one wing increases the lift, the opposite aileron does not, and will often work to decrease lift. Some aircraft use flaperons, which combine both the functionality of flaps and ailerons in a single control, working together to increase lift, but to slightly different degrees so the aircraft will roll toward the side generating the least lift. Flaperons were used by the Fairey Aviation Company as early as 1916 but didn't become common until after World War II.
Notes
- ^ Gunston, Bill, The Cambridge Aerospace Dictionary Cambridge, Cambridge University Press 2004, ISBN 978-0-521-84140-5/ISBN 0-521-84140-2 p.452
- ^ Toelle, Alan (2003). Windsock Datafile Special, Breguet 14. Hertfordshire, Great Britain: Albatros Productions, LTD.. ISBN 1-902207-61-0-1.
- ^ Gunston, Bill, The Cambridge Aerospace Dictionary Cambridge, Cambridge University Press 2004, ISBN 978-0-521-84140-5/ISBN 0-521-84140-2 p.584
- ^ Gunston, Bill, The Cambridge Aerospace Dictionary Cambridge, Cambridge University Press 2004, ISBN 978-0-521-84140-5/ISBN 0-521-84140-2 p.569
- ^ Smith, Apollo M. O. (1975). "High-Lift Aerodynamics" (PDF). Journal of Aircraft 12 (6): 518–523. ISSN 0021-8669. http://www.arvelgentry.com/amo/High-Lift_Aerodynamics.pdf. Retrieved 2011-07-12.
- ^ Gunston, Bill, The Cambridge Aerospace Dictionary, Cambridge, Cambridge University Press 2004, ISBN 978-0-521-84140-5/ISBN 0-521-84140-2 p.249-250
- ^ Flight 1942
- ^ National Aeronautics and Space Administration. Wind and Beyond: A Documentary Journey Into the History of Aerodynamics.
- ^ Gunston, Bill, The Cambridge Aerospace Dictionary, Cambridge, Cambridge University Press 2004, ISBN 978-0-521-84140-5/ISBN 0-521-84140-2 p.331
- ^ Gunston, Bill, The Cambridge Aerospace Dictionary, Cambridge, Cambridge University Press 2004, ISBN 978-0-521-84140-5/ISBN 0-521-84140-2 p.270
- ^ C.M. Poulsen, ed (July 27, 1933). ""The Aircraft Engineer - flight engineering section" Supplement to Flight". Flight Magazine: p.754a-d. http://www.flightglobal.com/pdfarchive/view/1933/1933%20-%200200.html.
- ^ NASA on High-Lift Systems
- ^ Virginia Tech – Aerospace & Ocean Engineering
- ^ Gunston, Bill, The Cambridge Aerospace Dictionary Cambridge, Cambridge University Press 2004, ISBN 978-0-521-84140-5/ISBN 0-521-84140-2 p.335
- ^ from German wiki page on Krüger flaps @ http://wikipedia.qwika.com/de2en/Kr%C3%BCgerklappe (accessed Oct 18, 2011)
- ^ Gunston, Bill, The Cambridge Aerospace Dictionary Cambridge, Cambridge University Press 2004, ISBN 978-0-521-84140-5/ISBN 0-521-84140-2 p.191
Bibliography
- Clancy, L.J. (1975), Aerodynamics, Chapter 6, Pitman Publishing Limited, London ISBN 0-273-01120-0
See also
|
|
General |
|
|
Military |
|
|
Accidents/incidents |
|
|
Records |
|
|